Adaptation
1. Introduction to Evolution (15 minutes)
We begin with a question: why are
there so many species of living thing? Children watch
a short film showing the wide variety of animal species
on earth - nearly 2 million known, and according to the latest estimate 8.7
million in all.
We discuss the idea that all living species have evolved over a vast period of
time: they have changed in order to adapt to their environments. This began
about 3.8 billion years ago with tiny bacteria and continues today. This
leads us to an astounding fact - all living things are related.
The first person to explain how evolution worked was Charles Darwin. This
workshop is all about the how and why of evolution - the tree of life,
adaptation, inheritance, and natural selection.
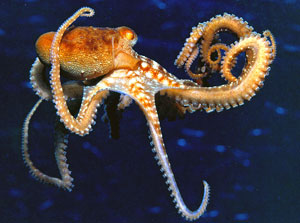
Next is a quick look at how animals are classified into groups - which themselves
have evolved. We look at a few important animal phyla - cnidarians
(jellyfish, corals, sea anemones), molluscs (squids,
shellfish, snails), arthropods (insects, spiders,
scorpions, centipedes), and vertebrates (fish, amphibians,
reptiles, mammals, birds). Each of these groups is very different from the
others, but all are related, although their common ancestors lived over 600
million years ago.
2. The Tree of Life (45 minutes)
We look at a large painted board (60x200cm, see
below) which shows a simplified tree of life - the branches
which show the relationships through time between the four phyla we have
already looked at, as well as the main branches of vertebrates (fish, birds,
mammals, etc. but also dinosaurs, ichthyosaurs, plesiosaurs, and primates).
The geological periods and their dates are also marked, going back 660 my
(my = million years). For a detailed page on
this topic go here.
Tree of Life game - click for larger version and see
picture below
To learn about the tree and the periods of earth's history, children
play a game: they are given a model of a prehistoric
animal such as a dinosaur or ammonite, as well as real fossils. They are
told
its age in millions of years, and they must place it on the board in the
right place - the right period and the right branch.
There follows a short quiz to enrich understanding - for example working out
the period in which a type of animal first
evolved (e.g. dinosaurs in the Triassic), or the ancestral history of
modern groups (e.g. humans are apes descended from primates, then from
mammals, reptiles, tetrapods, and fish).
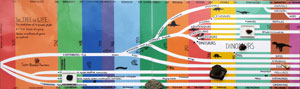
Finally children work in their 8 teams with their own tree
of life picture (click photo above). They must place 6 fossils
(dinosaur bone, trilobite, ammonite, shark tooth, coral, crocodile tooth -
see photo below) on
the tree in the right place, and answer some more questions about the tree.
This will enrich
their understanding of the history of evolution.
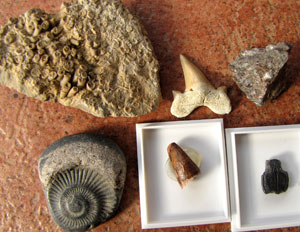
Click for larger version
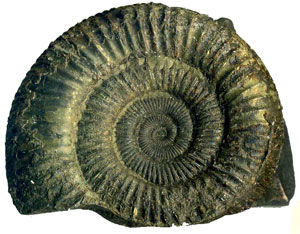
Ammonite, Dactylioceras commune, from Whitby: 190 my
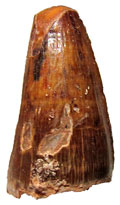
Trilobite from USA: 500 my, and crocodile tooth: 80 my
3. Adaptation (45+ minutes)
Why do species have their particular features? Why have birds evolved
feathers, and fish fins? We now look at adaptation
- the countless ways in which animals have changed through evolution to
survive and reproduce in their environments.
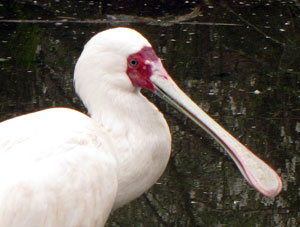
Spoonbill - it swishes its strange bill through the water, sensing and
grabbing food
First we watch 3 short films (which I have made myself). These illustrate
a few of the most important kinds of adaptation - for feeding, moving,
and sensing. After each film we discuss the various ways in
which animals have adapted for that purpose.
Then children (or the teacher) can choose two or three more activities to
learn about other types of adaptation.
Options:
a. Film about courtship and mating (including shots of
animals mating such as the damselflies below)
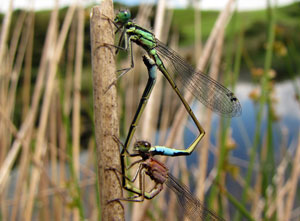
b. Film about raising babies
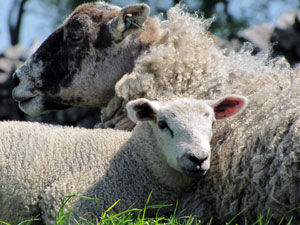
c. Camouflage game - look at photos and
try to spot the animal, whether predator or prey.
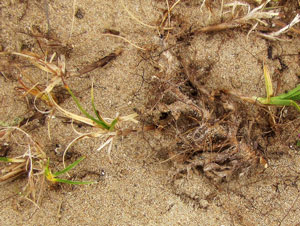
Spot the spider (click for larger version)
d. Mimicry game - look at photos of animals (butterflies,
frogs, wasps). Try to work out whether they belong to an unpalatable
(poisonous) species, or are palatable mimics which have evolved to look like
the unpalatable species, so as to avoid being eaten.
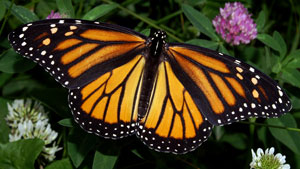
Monarch butterfly - tastes bad to predators
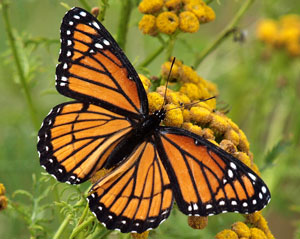
Is this a Monarch, or a Viceroy, which mimics the Monarch?
e. Evolution of Sight - watch a film to show how eyesight
evolved first in trilobites with simple compound eyes. The film shows how
the underwater world would have appeared, as a simple mosaic without colour.
Then compound eyes evolved with more resolution, as well as colour
perception, as we see in
modern insects.
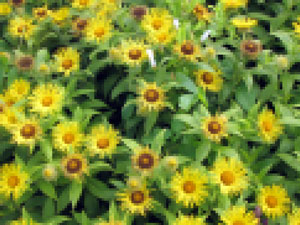
How flowers appear to a bee
We then
watch
a film and try an experiment - compare how a bee sees
the world (with 7000 lenses per eye) to how a dragonfly sees it (29,000 lenses
per eye). What sort of things can these two animals see? Why does the
dragonfly need so many lenses?
f. Evolution of Colour Vision - watch a short film to show
how animals at first saw without colour, and then how the world looked
different when colour vision evolved. Why would this be a useful adaptation?
At the time of the dinosaurs, mammals were nocturnal (to avoid being eaten!)
and so lost colour vision (which does not work at night and may actually
impair night vision). The film shows how a cat sees the world at night -
much more brightly than we do.
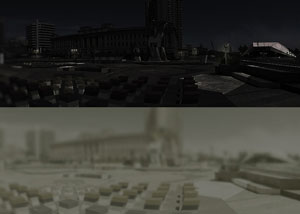
Night vision of a human (top) and cat (bottom)
Colour vision in primates
About 30 million years ago, primates regained full colour vision, which is
why we have it but most mammals do not. We try an experiment to try to
learn why this happened - comparing photos of fruit trees with fruit which
is red when ripe, and leaves which are red when younger and juicier.
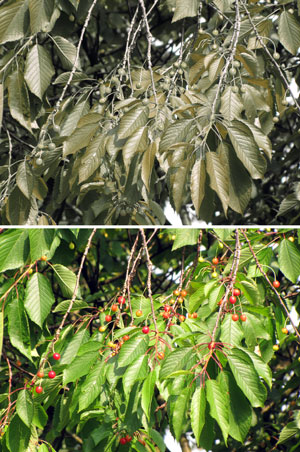
A cherry tree as a colour blind monkey would see it (top) and as we see it
(bottom) - click for larger version
If you want to do option 2 in the
Natural Selection workshop,
the simulation of how colour vision evolved in monkeys, then we will do this
colour vision activity.
4. Adaptation Model Activity (20-30 minutes)
In 8 small groups, children look at 4 models of animals or plants, with
information sheets provided, and identify some of their adaptations (e.g.
the long neck of a giraffe for reaching leaves). They discuss them in their
teams and present 1 or 2 of these animals to the class.
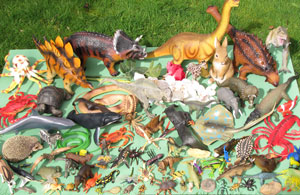
Some of the models used in the workshop - click for larger version
Lesson plan continues in the next column
→
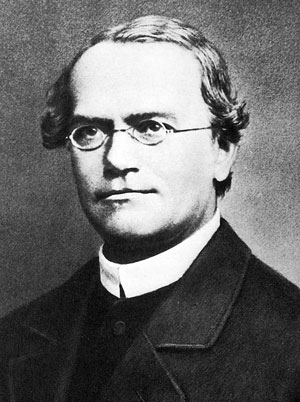
Gregor Mendel, 1822-1884, founder of modern genetics
|
5. Adaptation Activities (90 minutes
including explanation and discussion)
After lunch children do 5 activities in their 8 groups. They use models,
fossils, skulls, teeth and photos to enrich
their understanding of adaptation and start them thinking about
natural selection.
a. Habitat (16 minutes)
Children arrange 21 models of animals on a board according to their habitat (land,
air or water). They identify adaptations for living in these habitats, and draw conclusions about
the challenges of these environments. They also look at the relationship between size (ranging from ants to blue whales)
and habitat.
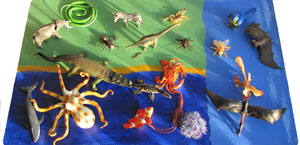
b. Camouflage Experiment (16 minutes)
Children reenact the famous case of the evolution of the peppered moth.
In the early 1800s this moth was a light, mottled colour (see below),
camouflaged against the lichen-covered bark of trees in England.
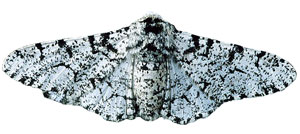
By the end of the 19th century, in areas like Manchester, this form of the
peppered moth had been almost entirely replaced by a darker mutant (see
below). The reason was that industrial pollution had darkened the trees, so
the lighter moths were no longer camouflaged and were being eaten by birds.
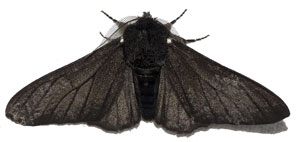
Children pretend to be the bird predator and try to spot the two
varieties of moth on light and dark tree backgrounds. By comparing the
numbers of moths seen, they should be able to work out how evolution
occurred in this situation. In the Natural Selection
workshop we return to this moth as a
famous example of evolution in action.
c. Transitions (16 minutes)
According to Darwin's theory, species evolve gradually, so there are
'transitional forms' along the branches taken by each type of animal.
Scientists have found many fossils of these transitional forms. One famous
case is of the various hominin species, such as
Australopithecus and Homo erectus, whose brain sizes and features such as
height and brow ridge show a gradual change over time. Another example is tetrapods
- the first four-legged animals - and the fish with leg-like fins which
preceded them, most famously Tiktaalik.
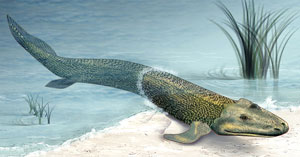
Tiktaalik, about 375 mya
Children learn about transitions by
ordering pictures of animals in 5
groups (tetrapods, dinosaurs, horses, whales, and humans). As an
extension for another day, you can print this activity:
children will make a graph of hominin brain size over the
last 3 million years, and try to draw the shape of a Homo erectus skull,
given drawings of Australopithecus and modern human skulls.
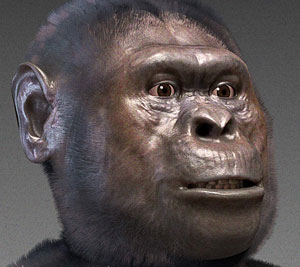
Australopithecus afarensis - one of our earliest human ancestors
(3.2 my)
d. Evolution of Birds (8 minutes)
Children examine 3 fossil replicas to learn about how
various animals are related to birds, and so how birds evolved from
dinosaurs. The fossils are Archaeopteryx (a famous transitional
fossil, a 150 my-old flying dinosaur with visible feathers but clear differences to birds such
as claws on its wings),
Compsognathus (a small dinosaur with a
skeleton similar to Archaeopteryx, and which lived at the same time) and a
bird (similar to a Rail,
from the Eocene period, 50 million years ago).
Archaeopteryx
Children look at features such as limbs, feathers
and claws and try to work out which fossil is which (given pictures of the
living animal). They then work out the order in which these animals are related
to birds, and so draw conclusions about how birds evolved, and why feathers
and wings might be useful adaptations.
e. Evolution of Teeth (8 minutes)
Children examine animal skulls and teeth, including
fossils,and arrange them first by diet (herbivore or carnivore), and then group (mammal
vs non-mammal), with pictures to help. They draw conclusions about what
sort of teeth evolved for what purpose. As an extension they can also work
out that only mammals
have differentiated teeth (canines, incisors and molars), and discuss why this might have evolved.
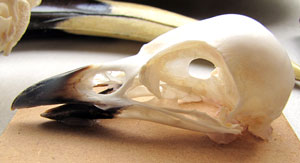
Skulls/teeth used: rabbit skull, fox skull, cat skull, shark
jaws, Triceratops tooth, Iguanadon tooth, magpie skull (above), Ichthyosaurus skull,
sabre-toothed cat tooth, mammoth tooth.
Click here to see them all.
At the end we will discuss children's
findings, and fossil prizes will be given for some of the activities.
Alien Evolution Game (30 minutes)
To finish we play a fun game. Each team chooses one
of 8
habitats and invents either a predator or prey animal, adapted both to its environment
and to other animals. For example a cheetah is the fastest four legged animal
with many adaptations to help it chase its prey, such as a very long and
flexible spine. However, its prey, the Thompson's gazelle, is also one of
the fastest animals on land and has evolved this speed to escape
cheetahs.
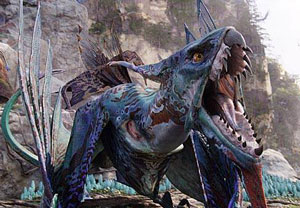
The twist in this game is that we imagine we have travelled
to another planet, so children can create brand new creatures - as long
as they are properly adapted (the creature above is from Avatar).
They will use marker pens and large sheets of paper to create their animals and present them to the class.
With fossil prizes for the best team.
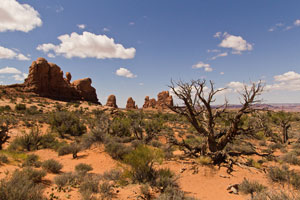
Desert habitat - others in the game are rainforest, savanna, ice cap,
mountains, woodland, wetland, and coral reef
Fossils for Sale
Over lunch or after school, fossils will be available for sale, at low prices
- mostly 10, 20 or 50p, plus a few for £1 or £2. I will also have dinosaur
and reptile toys for 50p-£2.
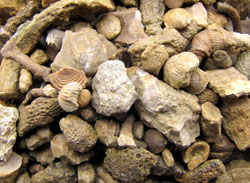
Corals and brachiopods
Natural Selection
The focus in this lesson is on the mechanism
of evolution - how does it actually work? We look at inheritance and
genes in the morning, then turn to natural
selection in the afternoon.
These vitally important ideas are difficult, even for adults,
so I have carefully crafted activities which will help children and teachers
get to grips with them. To bring the concepts to life, and make them more fun, we use photos, reenactments, dice, counters and cards, in a variety of
games and simulations.
1. Inheritance (morning session)
First we discuss inheritance - how we
resemble our parents both physically and in our behaviour and personality.
Game - identify children from their parents.
In 8 teams, children try to assign
photos of children to photos of
their parents (including both famous and non-famous families). We discuss how similar children are to their mothers and fathers, and why this
might be.
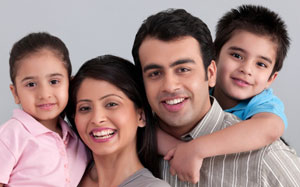
Genetics - we take a (simplified)
look at how inheritance works, by means of chromosomes and genes, which come in pairs
(called alleles).
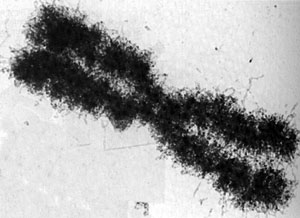
photo of a chromosome - the DNA strands are visible
Sex Chromosomes
We look at how X and Y chromosomes are inherited
to determine gender, using cards, counters and dice (see below). Children
learn why half the world's population is male and half female, and yet in
any one family there may be only boys or only girls - a matter of
probability. Each team randomly creates a family of four using dice and we
look at patterns across the class.
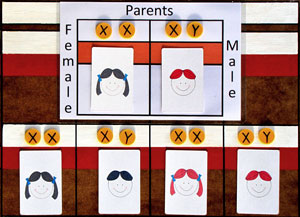
Genes
Next we focus on genes. Gregor Mendel's experiments on peas
showed us how traits are caused by indivisible factors called genes,
which come in pairs. One of the pair is often dominant - so
a pea flower with a purple gene (P) and a white gene (p) will end up purple,
as the purple gene is dominant.
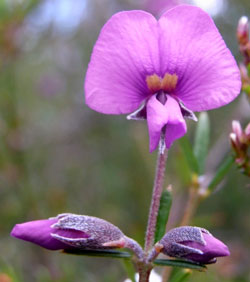
The genotype for this flower is either PP or Pp - both result in a purple
colour
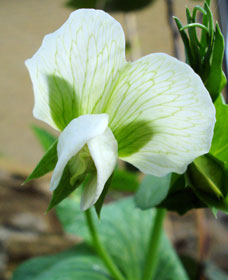
The genotype for this flower must be pp (two recessive genes)
Using gene cards and dice we look at how a plant or animal
inherits half of its genes from its mother and half from its father, but the
halves are randomly selected from all the pairs of alleles. This is why two
siblings can be very different from each other.
Optional Extra - Twins
If you wish we can look at twins,
and how identical twins share all their genes while non-identical twins
share about 1/2 (like ordinary siblings). This might be a good option if you
feel the full lesson on genetics is too difficult for your class (see later
section on cat colour).
Children play a game in which they sort 3
groups of photos - identical twins, non-identical twins, and unrelated
friends. This teaches them how physical appearance is largely inherited, and
how siblings can look very similar but also very different, because of the
random mix of genes they get from their mothers and fathers. Includes the
amazing case of the twins who appear to be of different races.
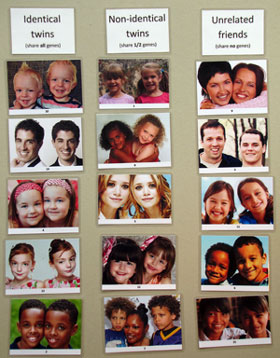
Lesson plan continues in next column
→
|
Inheritance lesson, continued:
Cat Fur Colour
For the rest of the morning session we look
at the inheritance of coat colour and pattern in cats. Cat colour is caused
by several genes, which combine to produce an end result (the 'phenotype').
For example, a cat with the dominant white spotting gene and one dominant
orange gene, if female, will usually be a white tortoiseshell (see below) -
usually, because other genes can modify this pattern.
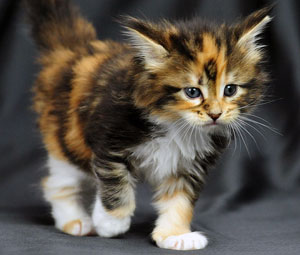
First we look at how the 'white spotting' gene (S) and the
'orange' gene (O) work separately. In each case after a demonstration
children work in their groups, first working out the genotype of cats
in photos, using counters instead of letters to help make the concept
concrete.
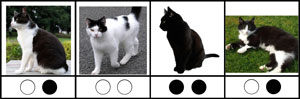
As we see in the first cat above, a cat with one dominant white spotting gene and
one recessive gene (Ss) will be less than half white (we assume the other
colour is black for now). A cat with two dominant genes (SS) will be mostly
white. A cat with two recessive genes will have no white (here, all black).
After this the teams use counters and cat phenotype cards to see what possible
kittens come from parents with
different genotypes. Children answer questions to help them grasp important
features of inheritance - namely, how parental genotypes affect:
-
the chance of different types of offspring
-
the chance of offspring being different from their
parents
-
the amount of variation in offspring
Next, we look at how the white and orange genes have a combined effect. Again children
solve photo puzzles. For example, a cat with Ss and Oo genes will be a
tortoiseshell with some white patches (the first cat below):
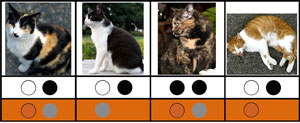
After this children are taught how to use gene counters and cards to lay out the 16 possible
kittens resulting from two parents. The photo below shows the board used by
each team - similar to the tables used by scientists to work out gene
combinations, known as Punnett Squares. Again teams answer questions about
inheritance and variation in offspring. There are fossil prizes for the team
which gets the highest score overall.
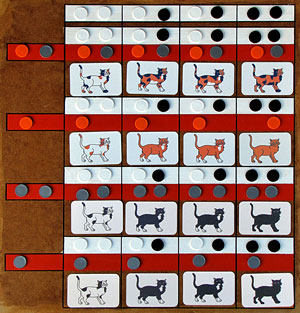
Mutation
The above activities show children how genes combine to
produce offspring which are similar to, but often different from, their parents -
and their siblings too. This variation due to recombination
of genes is part of what allows natural selection to take place. But
on its own, it is not enough.
Our genes have been passed down through millions of
generations, back to the earliest animals which walked on land, and the fish
which came before them (and all the way back to the first living thing). And
yet we are not fish! If genes are copied each generation, how can there be
so many different species? Where do new genes come from to create new
adaptations?
The answer is mutation. Very occasionally a
gene mutates, due to copying errors, radiation, or other reasons. Usually
this has no effect. Sometimes it is harmful, and the animal often dies
before passing the gene on. However, on rare occasions the mutant gene is
helpful - for example it may give a giraffe a longer neck. A mutation such
as this is what allows
natural selection to work.
2. Natural Selection (afternoon session)
Darwin's theory has been described as the greatest scientific idea of all time. In
principle it is quite simple - and yet most of us have a shaky
grasp of it. In this session children re-enact and simulate natural
selection through games, thus getting a concrete sense of how it works.
Peppered Moths - Evolution Observed
We return to the moth activity from the Adaptation workshop, looking
at the data from all 8 groups, to remind ourselves how camouflaged moths
were less likely to get eaten. In the early 1800s few dark moths were ever
seen. Most peppered moths rested on light, lichen-covered trees, and the black
ones tended to get eaten. By 1895, however, nearly all peppered moths found
near Manchester were black.
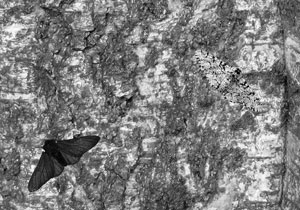
We discuss how, during the industrial revolution, trees
grew dark with soot. Children think about how this change might have
affected the moths.
To bring what happened to life, we carry out a reenactment.
Children hold pictures of light or dark moths, shown against the dark
background of the polluted bark. A bird (a child wearing a bird hat) 'eats'
moths - far more light ones than dark - and so we see how nature selects for darker, better camouflaged moths. The surviving moths
then reproduce, doubling their numbers. We repeat this for about 6 generations,
and see what happens.
Of course the genes for dark colour are passed on, and
so the numbers of dark moths increase while light moths diminish. In this way
we see how evolution
takes place.
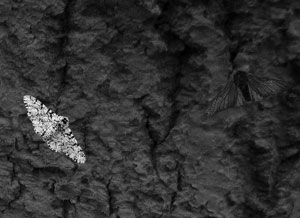
We discuss the experiment and try to reach an understanding of natural
selection. This process accounts for nearly all of evolution (other processes
also cause genetic change, but only natural selection
leads to adaptation).
Further Simulations
Children now run their own experiments in their groups, simulating a
real-life case of evolution by natural selection. There are 2 options,
depending on the ability of your children, involving simulations of
increasing complexity and accuracy in modelling the way genes are inherited.
Option 1 - Bacterial resistance followed by Peppered Moth
Resistance of bacteria to antibiotics is a major problem in the modern
world, with diseases such as MRSA claiming thousands of lives each year. In
this simulation we learn about bacteria, how they can cause disease
although many are helpful, how they reproduce and mutate very quickly, and
how this can lead to bacterial resistance if we misuse antibiotics.
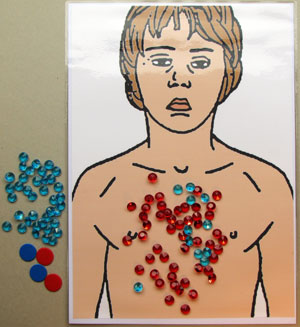
Children work in their 8 groups using red and blue gems and a picture
of a sick boy to simulate bacterial evolution. We see how infection leads to
sickness as bacteria multiply, and how antibiotics can kill off the bacteria
within a week or so. However if we do not complete the course of pills,
mutations can arise which make the bacteria resistant to medicine. They
multiply and can cause further problems - including spreading to other
people.
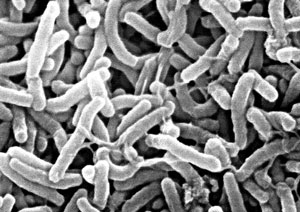
Cholera bacteria
It is also important not to take antibiotics when a disease is caused by a
virus, as with common cold and flu, because beneficial bacteria will be
killed, and the fast multiplication of survivors can lead to harmful mutations.
Peppered Moth
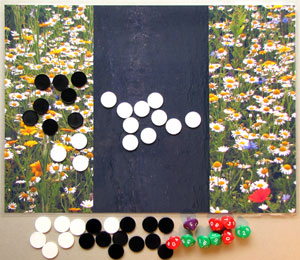
Having re-enacted the evolution of the peppered moth earlier, children will
be ready to take it a step further.
In this simulation we
introduce the random effects of survival, thus modelling natural
selection more accurately.
In their groups children use a large photo of a dark tree, black and
white counters, and 10-sided dice to carry out the simulation. We begin in
the mid 1800s with mostly light coloured moths - 15 white counters, and 1
mutant dark moth - a black counter. Some moths land on the tree and children
roll the dice to see if they survive. We assume light moths have a 40%
chance of not being eaten by a bird, and black moths have a 60% chance
because they are better camouflaged.
Each generation of survivors doubles its numbers, and the simulation
continues for as long as time allows. One child records the numbers of light
and dark moths in each generation and makes a graph.
If the task is completed and time is available, there is an extension. Children look at research done in the 20th century to test the
theory
that the peppered moth evolved due to differential bird predation. Children come up with their own experiment to test this idea.
Option 2 - Peppered Moth followed by Colour Vision in Monkeys
For the most authentic option, we first do the moth activity above, then the evolution of the primate colour vision gene.
This option is challenging and only suitable for children of above average
ability.
Children use gene cards and 10-sided dice to see how the gene for full
colour vision increases in a population over several generations. This
occurs because monkeys that can see ripe red
fruit or juicy leaves have a survival advantage over those that can't. We
assign numbers to this advantage - a 60% chance of survival (to
reproduction) of colour-sighted monkeys vs. a 40% chance for colour blind
monkeys.
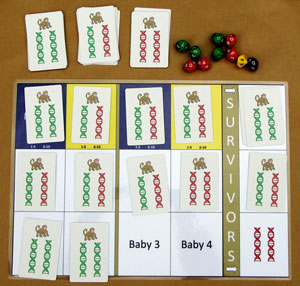
First, 'parent' monkey cards are dealt out. There are 2 alleles on each card
- a red and green picture of a length of DNA. Two greens means a colour
blind monkey; one or two reds means the monkey has full colour vision.
By rolling dice, children work out which gene each baby gets from its parents, and so put down the appropriate card (this is something they
have already practised with the gender cards - see above). They then roll
dice to see how many children of each genotype survive. After all the
parents and babies in the current generfation have been dealt and rolled
for, children count the survivors of each genotype and record the data. One
child makes a graph to show how the percentage of colour-sighted monkeys
changes.
This process is repeated for as many generations as time allows (about 6).
Eventually it will become clear to children that the number of genes
for colour vision is increasing. This will help them understand how both natural selection and genetic inheritance
work.
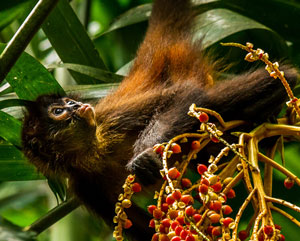
Summary and Final Activity
For both options we finish with a discussion of what we have learned from our
simulations, and see if we can come up with a definition for natural
selection.
Finally children apply the idea of natural selection to
another animal - for example, how brain size might have evolved in humans,
big ears in elephants, or nest building in birds. Each team discusses a
case and presents it to the class, with fossil prizes for the best presentation.
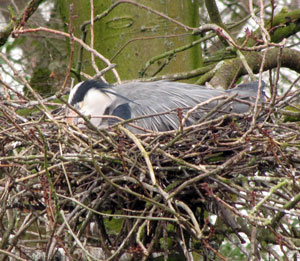
|